If your slow Internet connection has you frustrated, just be glad you don't have to communicate with a probe on Mars. Even with new technology, scientists will be able to transmit only about 270 megabits per second between the two planets, not a lot of information when you're talking about superhigh-resolution photos. But a new study suggests that by twisting laser light, scientists could pack enough information into interplanetary beams to speed up extraterrestrial communications to the multi-gigabit level.
At the moment, laser-based communication between Earth and satellites uses so-called pulse-position modulation (PPM). In this strategy, information is coded into the timing of an infrared laser pulse. A given time span is broken into short windows, and data is encoded by sending the laser light to a detector during a particular window. To encode the alphabet, for example, the time span could be broken into 26 windows, one for each letter.
You could get higher bandwidth by cramming more windows into each time span. But the faster, more complex electronics needed to do that would make for an expensive and power-hungry system, says Ivan Djordjevic, an optical communications expert at the University of Arizona, Tucson. Instead, he says, we should scrap time encoding and harness a different property of light: its orbital angular momentum (OAM), a twisting pattern in the beam.
OAM concerns the phase of the beam, or where it is in its oscillation between two peaks of the wave. Swaths of the beam are in the same place in their oscillation, and they are said to have the same phase. If light has no OAM, these same-phase regions cut across the beam, like the broad crest of an ocean wave approaching the shoreline. But in light with OAM, the regions with the same phase twist instead around the direction of travel in spiraling ribbons.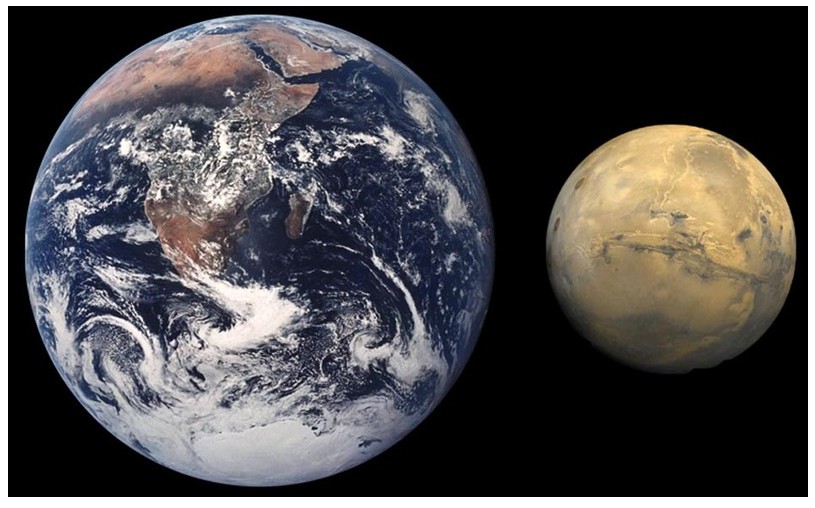
The more OAM, the more complex the pattern, forming single, double, and triple helices on up. These helical shapes are handy for encoding data. Whereas an untwisted light beam can represent two symbols (say, 0 or 1) depending on whether it is turned off or on, light that comes in any combination of three helices can represent 23, or eight, symbols (say, 0 through 7). By giving each wave four different intensity options instead of two, you can increase the vocabulary of a single pulse to 43, or 64, possible symbols.
The combination of different shapes and intensities can encode more information into a single pulse than is practical through pulse-position modulation, Djordjevic says. He argues that the equipment needed to encode and decode OAM waves can be built by low-cost upgrades to current technologies. The pulses would be sent by a laser and passed through a hologram or multimode optical fiber, which twists the light. On the other side, a telescope would focus the light and a second hologram, or fiber would decode the signal.
One possible stumbling block for the technique is that Earth's atmosphere bends light differently as it warms or cools, scrambling OAM signals. But in a paper in the 18 July issue of Optics Express, Djordjevic reports that computer simulations showed that it is possible to reconstruct the original OAM signal. If the turbulence isn't particularly strong, this method can untangle up to nine different OAM states, he says.
Closer to home, Djordjevic suggests that OAM could also provide Internet links of 100 gigabits per second through the air. Data rates between satellites and Earth, between satellites, and even between satellites and aircraft could get a boost from harnessing twisted light. "Standard telescopes are sufficient for these short distances," he says.
Experts are cautious about the advance. "It is one of those things that works well in theory," says Hamid Hemmati, a laser communications expert at NASA's Jet Propulsion Laboratory in Pasadena, California. However, he and others point out a practical hurdle: Unlike simpler PPM pulses, which can be interpreted with small amounts of the original beam, a telescope has to capture most of an OAM beam to see its twisted structure. The trouble is that beams spread out as they travel. Hemmati and his colleagues estimate that receiving OAM data from a transmitter as distant as the sun would require a kilometer-wide telescope.
Not so, Djordjevic says. Telescopes that pick out light's polarization, another form of twisting, are estimated to need lenses only tens of meters across rather than hundreds. As both twists turn around light's direction of travel, he believes an OAM telescope shouldn't be any bigger.
Hemmati acknowledges that both sides are guessing. "We need to spend more time evaluating it," he says.